Healthcare Professionals
Resources for Health Professionals
On the Resources section of our site you can learn more about our research and publications.
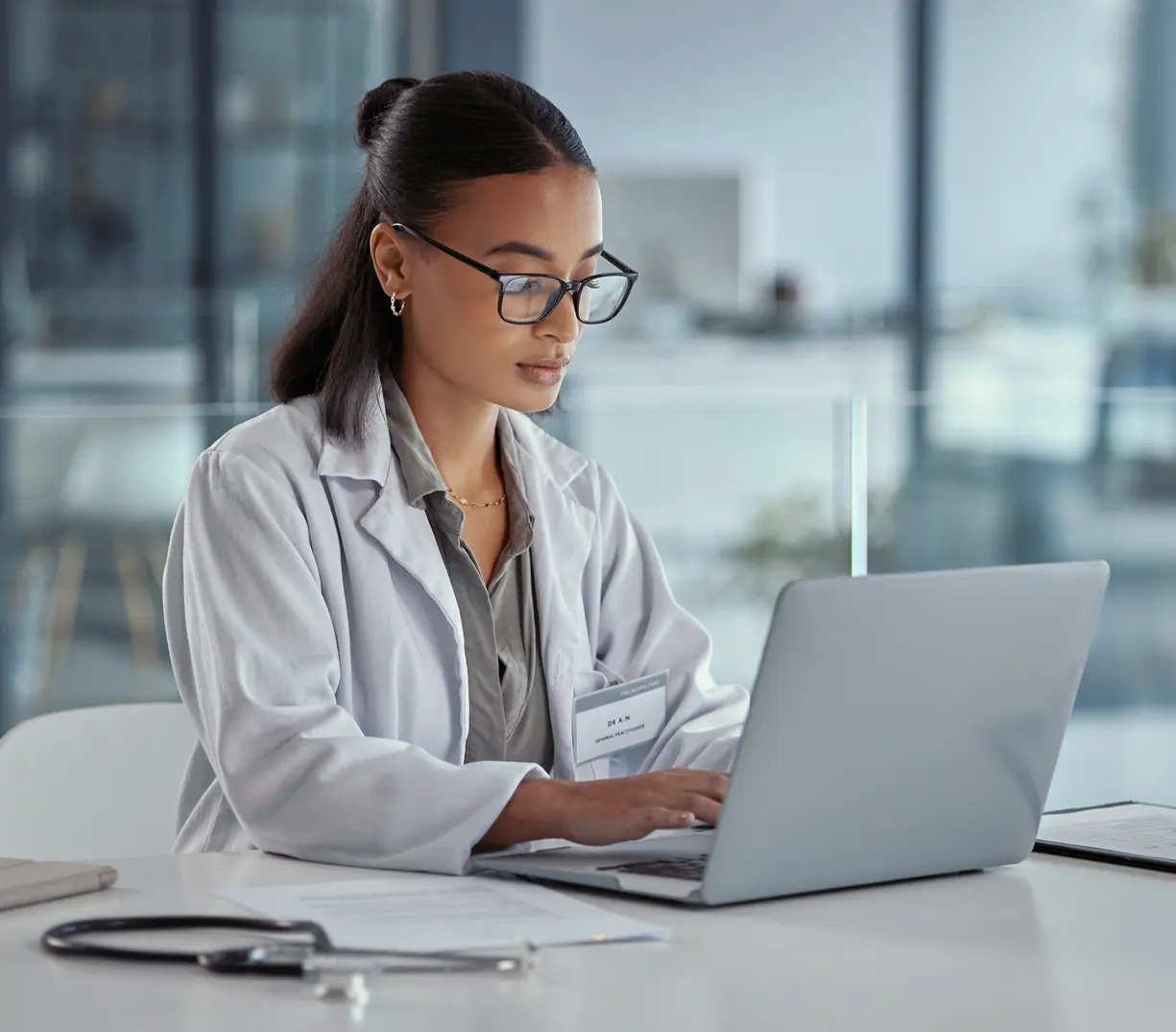
Noninvasive monitoring of the autonomic nervous system and hemodynamics of patients with blunt and penetrating trauma.
Colombo J, Shoemaker WC, Belzberg H, Hatzakis G, Fathizadeh P, Demetriades D.
Hemodynamic and oxygen transport patterns after head trauma and brain death: implications for management of the organ donor.
Belzberg H, Shoemaker WC, Wo CC, Nicholls TP, Dang AB, Zelman V, Gruen JP, Berne TV, Demetriades D.
Effects of age and obesity on hemodynamics, tissue oxygenation, and outcome after trauma.
Belzberg H, Wo CC, Demetriades D, Shoemaker WC.
Hemodynamic patterns preceding circulatory deterioration and death after trauma.
Chien LC, Lu KJ, Wo CC, Shoemaker WC.
Effects of age and obesity on hemodynamics, tissue oxygenation, and outcome after trauma.
Belzberg H, Wo CC, Demetriades D, Shoemaker WC.
A mathematical program to predict survival and to support initial therapeutic decisions for trauma patients with long-bone and pelvic fractures.
Lu K, Shoemaker WC, Wo CC, Lee J, Demetriades D.
Outcome prediction by a mathematical model based on noninvasive hemodynamic monitoring.
Shoemaker WC, Wo CC, Lu K, Chien LC, Bayard DS, Belzberg H, Demetriades D, Jelliffe RW.
Hemodynamic patterns of blunt and penetrating injuries.
Lu KJ, Chien LC, Wo CC, Demetriades D, Shoemaker WC.
Evaluation of invasive and noninvasive hemodynamic monitoring in trauma patients.
Shoemaker WC, Wo CC, Chien LC, Lu K, Ahmadpour N, Belzberg H, Demetriades D.
Noninvasive hemodynamic monitoring for combat casualties.
Shoemaker WC, Wo CC, Lu K, Chien LC, Rhee P, Bayard D, Demetriades D, Jelliffe RW.
Survival, hemodynamics, and tissue oxygenation after head trauma.
Nicholls TP, Shoemaker WC, Wo CC, Gruen JP, Amar A, Dang AB.
Outcome prediction in chest injury by a mathematical search and display program.
Shoemaker WC, Bayard DS, Wo CC, Chan LS, Chien LC, Lu K, Jelliffe RW.
Survival, hemodynamics, and tissue oxygenation after head trauma.
Nicholls TP, Shoemaker WC, Wo CC, Gruen JP, Amar A, Dang AB.
Outcome prediction in chest injury by a mathematical search and display program..
Shoemaker WC, Bayard DS, Wo CC, Chan LS, Chien LC, Lu K, Jelliffe RW.
A stochastic control program to predict outcome and to support therapeutic decisions: a preliminary report.
Shoemaker WC, Bayard DS, Wo CC, Botnen A, Ahmedpour N, Gandhi A, Demetriades D, Jelliffe RW.
A stochastic control program to predict outcome and to support therapeutic decisions: a preliminary report.
Shoemaker WC, Bayard DS, Wo CC, Botnen A, Ahmedpour N, Gandhi A, Demetriades D, Jelliffe RW.
The effect of obesity on bioimpedance cardiac index.
Brown CV, Martin MJ, Shoemaker WC, Wo CC, Chan L, Azarow K, Demetriades D.
Is noninvasive hemodynamic monitoring appropriate for the elderly critically injured patient?
Brown CV, Shoemaker WC, Wo CC, Chan L, Demetriades D.
Value of non-invasive continuous hemodynamic monitoring system in the differential diagnosis of the patients with dyspnea.
Gu ZL, Cheng CE, Wu G, Zhang BS, Lu ZK, Xu LH.
Application of non-invasive hemodynamic monitoring on high-risk surgical patients in the early stages after emergency admission.
Lu JQ, Li CS, Yang JY, Zhu J, Wo C, Shoemaker W.
Outpatient cardiovascular management utilizing impedance cardiography.
Lasater M, Von Rueden KT.
Bench to bedside: electrophysiologic and clinical principles of noninvasive hemodynamic monitoring using impedance cardiography.
Summers RL, Shoemaker WC, Peacock WF, Ander DS, Coleman TG.
Department of Emergency Medicine, University of Mississippi Medical Center, Jackson 39216, USA.
Meta-analysis of hemodynamic optimization in high-risk patients.
Kern JW, Shoemaker WC.
USC School of Pharmacy, Los Angeles, CA, USA.
New approaches to trauma management using severity of illness and outcome prediction based on noninvasive hemodynamic monitoring.
Shoemaker WC.
Department of Anesthesia and Surgery, Los Angeles County and University of Southern California Medical Center, University of Southern California.
Modeling Population Pharmacokinetics of Lidocaine: Should Cardiac Output Be Included as a Patient Factor?
Kuipers, J A.; Boer, de Roode, A,Olofsen, E; Bovill, JL. Burm, A G.
Orthostatic Changes in Bioimpedance Parameters Identify Decompensated Heart Failure in Emergency Department Patients.
W. Frank Peacock, Charles E. Emmerman, Brian A. Janz, Vidya Ramanavarapu, Philippine Kies.
Emergency Medicine, The Cleveland Clinic, Cleveland, OH.
Outcome Prediction of Emergency Patients by Noninvasive Hemodynamic Monitoring.
William C. Shoemaker, MD; Charles C. J. Wo, BS; Linda Chan, PhD; Emily Ramicone, MS; Eman S. Kamel, MD; George C. Velmahos, MD, PhD and Howard Belzberg, MD, FCCP
* From the Departments of Anesthesia (Dr. Shoemaker) and Surgery (Drs. Shoemaker, Wo, Kamel, Velmahos, and Belzberg), Los Angeles County/USC Medical Center; Division of Biostatistics and Outcome Assessment (Dr. Chan and Ms. Ramicone), University of Southern California, Los Angeles, CA.
Development of a Hemodynamic Database in Severe Trauma Patients to Define Optimal Goals and Predict Outcome.
WcC Shoemaker, CCJ Wo, A Botnen, RW Jelliffe.
University of Southern California Ds Bayard, Jet Propulsion Laboratory.
Endpoints of resuscitation of critically injured patients: normal or supranormal? A prospective randomized trial.
Velmahos GC, Demetriades D, Shoemaker WC, Chan LS, Tatevossian R, Wo CC, Vassiliu P, Cornwell EE 3rd, Murray JA, Roth B, Belzberg H, Asensio JA, Berne TV.
Department of Surgery, Division of Trauma and Critical Care, and the Department of Biostatistics and Outcomes Research, University of Southern California, Los Angeles, California, USA.
Noninvasive hemodynamic monitoring for early warning of adult respiratory distress syndrome in trauma patients.
Tatevossian RG, Shoemaker WC, Wo CC, Dang AB, Velmahos GC, Demetriades D.
Department of Surgery, University of Southern California and the LAC+USC Medical Center, Los Angeles, USA.
Invasive and noninvasive haemodynamic monitoring of acutely ill sepsis and septic shock patients in the emergency department.
Shoemaker WC, Wo CC, Yu S, Farjam F, Thangathurai D.
Department of Emergency Medicine, King-Drew Medical Center, Los Angeles, CA, USA.
Bioimpedance monitoring: better than chest x-ray for predicting abnormal pulmonary fluid?
Peacock WF IV, Albert NM, Kies P, White RD, Emerman CL.
Cleveland Clinic, Cleveland, OH.
Cardiac and hemodynamic assessment of patients with cocaine-associated chest pain syndromes.
Baumann BM, Perrone J, Hornig SE, Shofer FS, Hollander JE.
Hospital of the University of Pennsylvania, Philadelphia 19102, USA.
Randomized, double-blind, placebo-controlled trial of diazepam, nitroglycerin, or both for treatment of patients with potential cocaine-associated acute coronary syndromes.
Baumann BM, Perrone J, Hornig SE, Shofer FS, Hollander JE.
Department of Emergency Medicine, Hospital of the University of Pennsylvania, Philadelphia 19102, USA.
Early continuous noninvasive hemodynamic monitoring after severe blunt trauma.
Velmahos GC; Wo CC; Demetriades D; Shoemaker WC.
Department of Surgery, University of Southern California School of Medicine, Los Angeles, USA.
Hemodynamic patterns of survivors and nonsurvivors during high risk elective surgical operations.
Shoemaker WC, Wo CC, Thangathurai D, Velmahos G, Belzberg H, Asensio JA, Demetriades D.
Department of Surgery, University of Southern California School of Medicine, 1200 N. State Street, Room 9900, Los Angeles, California 90033, USA.
Intraoperative evaluation of tissue perfusion in high-risk patients by invasive and noninvasive hemodynamic monitoring.
Shoemaker WC; Thangathurai D; Wo CC; Kuchta K; Canas M; Sullivan MJ; Farlo J; Roffey P; Zellman V; Katz RL
Department of Anesthesiology, University of Southern California School of Medicine, Los Angeles 90033, USA.
Advances in continuous, noninvasive hemodynamic surveillance. Impedance cardiography.
Von Rueden KT, Turner MA.
Invasive and non-invasive physiological monitoring of blunt trauma patients in the early period after emergency admission.
Velmahos GC; Wo CC; Demetriades D; Murray JA; Cornwell EE 3rd; Asensio JA; Belzberg H; Shoemaker WC.
Department of Surgery, University of Southern California, Los Angeles, USA.
Physiologic monitoring of circulatory dysfunction in patients with severe head injuries.
Velmahos GC, Shoemaker WC, Wo CC, Demetriades D.
Department of Surgery, Division of Trauma and Critical Care, University of Southern California and the Los Angeles County/USC Medical Center, 1200 N. State Street, Room 9900, Los Angeles California 90033, USA.
Invasive and noninvasive hemodynamic monitoring of patients with cerebrovascular accidents.
Velmahos GC; Wo CC; Demetriades D; Bishop MH; Shoemaker WC.
Department of Surgery, Los Angeles County-University of Southern California (LAC-USC) Medical Center 90033, USA.
Multicenter study of noninvasive monitoring systems as alternatives to invasive monitoring of acutely ill emergency patients.
Shoemaker WC; Belzberg H; Wo CC; Milzman DP; Pasquale MD; Baga L; Fuss MA; Fulda GJ; Yarbrough K; Van De Water JP; Ferraro PJ; Thangathurai D; Roffey P; Velmahos G; Murray JA; Asensio JA; El Tawil K; Dougherty WR; Sullivan MJ; Patil RS; Adibi J; James CB; Demetriades D.
Department of Surgery, University of Southern California School of Medicine, Los Angeles, USA.
Continuous intraoperative noninvasive cardiac output monitoring using a new thoracic bioimpedance device.
Thangathurai D; Charbonnet C; Roessler P; Wo CC; Mikhail M; Yoahida R; Shoemaker WC.
Department of Anesthesiology, University of Southern California School of Medicine, Los Angeles, USA.
Oxygen transport and oxygen metabolism in shock and critical illness. Invasive and noninvasive monitoring of circulatory dysfunction and shock.
Shoemaker WC.
Department of Anesthesiology, University of Southern California School of Medicine, Los Angeles, USA.
Intraoperative maintenance of tissue perfusion prevents ARDS. Adult Respiratory Distress Syndrome.
Thangathurai D, Charbonnet C, Wo CC, Shoemaker WC, Mikhail MS, Roffey P, Roessler P, Kuchta K, Zelman V, DeMeester TR, Katz R.
Department of Anesthesiology, University of Southern California School of Medicine, Los Angeles, USA.
Early physiologic patterns in acute illness and accidents: toward a concept of circulatory dysfunction and shock based on invasive and noninvasive hemodynamic monitoring.
Shoemaker WC; Wo CC; Demetriades D; Belzberg H; Asensio JA; Cornwell EE; Murray JA; Berne TV; Adibi J; Patil RS.
Department of Surgery, University of Southern California School of Medicine, Los Angeles 90033, USA.
Temporal physiologic patterns of shock and circulatory dysfunction based on early descriptions by invasive and noninvasive monitoring.
Shoemaker WC.
Department of Surgery, University of Southern California School of Medicine, Los Angeles, USA.
Noninvasive hemodynamic monitoring of critical patients in the emergency department.
Shoemaker WC; Wo CC; Bishop MH; Thangathurai D; Patil RS.
Department of Emergency Medicine, King-Drew Medical Center, Los Angeles, CA, USA.
Noninvasive physiologic monitoring of high-risk surgical patients.
Shoemaker WC, Wo CC, Bishop MH, Asensio J, Demetriades D, Appel PL, Thangathurai D, Patil RS.
Department of Emergency Medicine, King-Drew Medical Center, University of Southern California School of Medicine, Los Angeles, USA.
Application of an artificial intelligence program to therapy of high-risk surgical patients.
Patil RS, Adibi J, Shoemaker WC.
Information Science Institute, University of Southern California School of Medicine, Los Angeles, USA.
Noninvasive cardiac index monitoring in gunshot wound victims.
Bishop MH; Shoemaker WC; Shuleshko J; Wo CC.
Department of Surgery, King-Drew Medical Center, Los Angeles, CA 90059, USA.
Invasive and noninvasive monitoring for early recognition and treatment of shock in high-risk trauma and surgical patients.
Asensio JA; Demetriades D; Berne TV; Shoemaker WC.
Department of Surgery, Los Angeles County/University of Southern California Medical Center, USA.
Resuscitation from severe hemorrhage.
Shoemaker WC, Peitzman AB, Bellamy R, Bellomo R, Bruttig SP, Capone A, Dubick M, Kramer GC, McKenzie JE, Pepe PE, Safar P, Schlichtig R, Severinghaus JW, Tisherman SA, Wiklund L.
Department of Emergency Medicine, King/Drew Medical Center, Los Angeles, CA 90059, USA.
Time-frequency distribution technique in biological signal processing.
Wang X, Sun HH, Van de Water JM.
Department of Electrical and Computer Engineering, Drexel University, Philadelphia, PA 19104.
Impedance cardiography in the measurement of cardiac output: studies in rabbits.
Pappas CG, Wang X, Connolly RJ, Schwaitzberg SD.
Department of Surgery, New England Medical Center, Boston, Massachusetts 02111, USA.
An advanced signal processing technique for impedance cardiography.
Wang X, Sun HH, Van de Water JM.
Department of Electrical and Computer Engineering, Drexel University, Philadelphia, PA 19104
Multicenter trial of a new thoracic electrical bioimpedance device for cardiac output estimation.
Shoemaker WC; Wo CC; Bishop MH; Appel PL; Van de Water JM; Harrington GR; Wang X; Patil RS.
Department of Emergency Medicine, King/Drew Medical Center, Los Angeles, CA 90059.
Cardiovascular changes during laparoscopic cholecystectomy.
Westerband A, Van De Water J, Amzallag M, Lebowitz PW, Nwasokwa ON, Chardavoyne R, Abou-Taleb A, Wang X, Wise L.
Department of Surgery, Long Island Jewish Medical Center.
An impedance cardiography system: a new design.
Wang XA, Sun HH, Adamson D, Van de Water JM.
Department of Electrical and Computer Engineering, Drexel University, Philadelphia, PA 19104